Editor’s note: PLAN-Boulder County has issued a report entitled Does Dense Make Sense? This is the third part in a six part series extracted from the report.
As the world strives to reduce greenhouse gas (GHG) emissions, we face a conundrum: whereas we all have to reduce our individual carbon footprints, the climate is sensitive to the total GHG content of the atmosphere. As cities like Boulder work to make progress toward Kyoto goals (8% below 1990 emission levels by 2012) and beyond, we need to reduce total carbon footprint regardless of changes in population. Fortunately, Boulder’s population has been growing at a rate slightly below 1% and we have a willing and dedicated citizenry that has indicated its support for climate action by passing the Climate Action Tax and the ClimateSmart loan program. Nonetheless, our population has grown by approximately 20,000 since 1990, or about 28%, which adds to the intensity of per capita reductions that are required. Additional population growth, even at our relatively slow current rate, which could double our population in 70 years, will make it that much more difficult to achieve Kyoto goals and goals defined by the Governor’s Energy Office (20% reduction from 2005 levels by 2020 and 80% by 2050). Fortunately, the City of Boulder is projected to reach “buildout” in 2035 with a population of 119,400, but other Boulder County communities need to seriously consider the GHG consequences of growth.
In this section, we examine the impact of the built environment on carbon footprints (GHG emissions). Much has been written in recent years about potential advantages of dense housing in reducing per capita GHG emissions due to close, convenient availability of mass transit, insulating effects of common walls in multiunit housing, and the heat island effect that warms ambient air around dense developments (Haughey, 2005; Ewing, et al. 2008). Lower per capita GHG’s in densely populated areas were illuminated when local and regional monitoring of atmospheric emissions as part of air quality studies were subjected to GIS analysis. These studies (Gurney et al., 2009) show, predictably, that major cities put out very high net greenhouse gases and pollutants (Figure 1), but a surprising outcome of these studies is that per capita emissions are noticeably lower than in rural areas (Figure 2). Estimates of total per capita GHG reductions vary widely, however, ranging from 20-40% (Ewing et al.) to 70% (http://www.cnt.org/). This extreme range suggests that methodology is still evolving, that estimation of carbon footprint for an area is a complex endeavor, and that careful examination of individual factors that affect GHG’s is warranted.
Figure 1. Total CO2 emissions in the U.S.
Source: http://www.purdue.edu/eas/carbon/vulcan/index.phpFigure 2. Per capita CO2 emissions in the U.S.
Source: http://www.purdue.edu/eas/carbon/vulcan/index.phpTransportation
We have already seen that denser housing concentrated around transit centers offers advantages in reducing vehicle miles traveled and especially single occupant vehicle usage. Both the Transportation Research Board (2009) report and Ewing et al. indicate that dense housing development offers a savings in vehicle miles traveled of 7-12%. This translates directly to a similar reduction in GHG’s due to transportation advantages. Greater savings may ensue if additional transit-friendly programs such as managed parking and convenient bus-train-bike-pedestrian facilities are offered.
Energy and Land Use Patterns
Attached housing offers some advantages in reducing GHG’s related to home heating and electricity usage. Ewing and Rong used data from the U.S. Residential Energy Consumption Survey, house types from the Census Bureau’s Public Use Microdata Sample, and house size statistics from the Census Bureau to conclude that nationwide housing in compact development results in less energy use and GHG emissions by 20-40% relative to detached housing. This is due to:
- Less heat loss from shared walls and ceilings
- The urban heat island effect, which causes a higher ambient temperature in dense areas and this slightly reduces heating costs (see footnote 1)
- Larger houses in detached housing areas
However, Randolph (2008) offers the following comment on Ewing and Rong, “Their complex methodology linking three unrelated data sets renders their quantitative conclusions suspect. Further, a simple engineering analysis can show more meaningful results, sprawl is more likely to affect energy use through increased vehicle miles traveled than house size or type, and household energy use can be mitigated by increasing the efficiency of the building envelope, heating/cooling system, appliances, and lighting.”
Energy Modeling
In order to determine efficiencies gained from multi-family housing, PLAN-Boulder County conducted an engineering analysis for comparable 1,040 square foot (sf) houses in attached and detached settings (see footnote 2). A single-family, 26×40, 1040 sf ranch house in Boulder was modeled and compared to a 12-unit, 3 story apartment building (1040 sf/unit) with additional area added for corridor, stairs and elevator. Both the house and the apartment building were designed with 2×6 frame walls at R19 insulation, R38 roof insulation, slab on grade with 2” perimeter insulation, low E insulated glass, and low air infiltration. The results are shown below, in BTU’s per hour.
Table 1. Relative Heat Loss and Heat Gain Values for Hypothetical Boulder Dwellings (BTUH)
House | Apartment Building | ||
Total Value | Individual Unit Value | ||
Heat Loss | 19,140 | 86,954 | 7,246 |
Heat Gain | 11,708 | 65,693 | 5,474 |
The combined heat loss and heat gain values for the detached house were 2.43 times greater than the apartment unit, which translates to 59% less energy use.
These energy-demand differences will vary due to climate/location, building size, building construction and solar orientation but provide a good representation of comparative energy-demand for this specific example, which is typical of housing types in Boulder, Colorado.
The difference between the Ewing and Rong results, which were statistically derived from national surveys of housing stock, and the direct engineering analysis for similarly constructed buildings, is due to differences in their data sets and methodologies.
Embodied Energy
Embodied energy is the energy required to construct a building, including the manufacture and operation of equipment and materials, extraction of raw materials, and transport of those materials to the building site. The University of Strathclyde Energy Systems Research Unit offers this example, “Basically, [embodied energy] means all the energy required to make a material, such as a clay brick. This includes the energy to extract the clay, transport it to the brick-works, mould the brick, fire it in the kiln, transport it to the building site and put the brick into place. It also includes all the indirect energy required, i.e., all the energy required to manufacture the equipment and materials needed to manufacture a brick, e.g. trucks, kilns, mining equipment, etc. All have a proportion of their energy invested in that brick.”
The Australian Commonwealth Scientific and Industrial Research Organization has found that the average house contains about 1,000 giga-joules (GJ) of energy embodied in the materials used in its construction. This is equivalent to about 15 years of conventional, operational, energy use. For an average house’s 20-year service life ,the embodied energy would be equivalent to 75% of its lifetime operational cost. Embodied energy can’t be ignored.
In Asia’s Alarming Cities (Banyan, Economist July 3, 2010) regarding urbanization in China, the author states, “In theory, urban living can be greener than other ways of life: people need to travel shorter distances, for instance. The practice is not so simple. Most striking, putting up and using buildings accounts for a big part of developing Asia’s carbon emissions — perhaps 30% in the case of China.”
According to the American Institute of Architects (Buildings, Oct 2008), the majority of existing public buildings have a lifespan of 50-100 years and embodied energy is as much as 10-15% of the energy used in a 100 year lifespan. Non-public buildings typically have much shorter lifespans and the embodied energy is consequently a greater portion of their overall energy impact.
The U.S. Energy Information Administration (EIA) states that buildings are responsible for almost half (48%) of all energy consumption and GHG emissions annually in the U.S. The U.S. Energy Research and Development Administration states that the annual embodied energy of building materials and the energy used in the U.S. to construct buildings is estimated at 1.146 MBTU/sf of building for new construction and half of this for renovation. At the current rate of construction in the U.S. of approximately 5 billion square feet (Bsf) of new building and 5 Bsf of renovation (EIA and Architecture 2030), the total annual energy consumed is approx. 8.6 QBTU, or 8.6% of total U.S. annual energy consumption. However, embodied energy for tall buildings is significantly greater than modest height buildings according to the Council on Tall Buildings and Urban Habitat.
Externally Produced GHGs
Dense urban developments draw on goods and materials from a large hinterland, and those GHG contributions must be considered. The GHG budget of a city or an area includes carbon output due to air travel (by far the most intense form of travel) and other forms of travel to and from cities, production and transportation of food and goods, the embodied energy of materials and supplies, and finally the GHG produced by trash disposal. These important factors have until recently been largely ignored. Ramaswami, et al. (2007) have produced more comprehensive estimates of the carbon footprint of metropolitan areas that include many of the factors listed above. They find that roughly 1/4 of CO2 emissions are not accounted for when carbon footprint calculations do not include external transportation and embodied energy considerations. Most importantly, life cycle calculations of energy and materials produced outside cities and consumed in cities need to be calculated for all cities and included in GHG inventories.
Figure 3. Denver’s GHG emissions summary by activity in 2005
(The hatched regions show sectors and activities typically not included in conventional city-scale boundary-limited direct GHG emissions inventories)
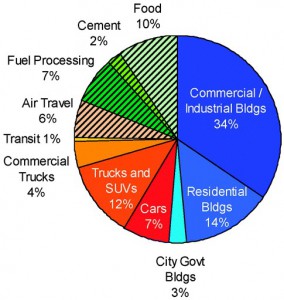
Peak Oil and High-rise Buildings in Very Dense Metropolitan Areas
Construction of dense housing that requires air conditioning and buildings that are too tall for walkups (generally taller than 5-6 floors) result in increased energy usage and may become extremely vulnerable in the post-oil world.
Numerous authors have predicted that we are at or near the peak of world oil and gas production (Deffeyes, 2001; Heinberg, 2004). The Association for the Study of Peak Oil and Gas (ASPO) provides up-to-date estimates of the current energy resource production.
Figure 4. Global Oil and Gas Production Profiles
Source: www.aspo-ireland.orgASPO’s latest model suggests that regular conventional oil reached an all time peak in 2005. If heavy oil, deep-water, polar and natural gas liquids are considered (the ‘all-liquids’ category), the model suggests that this peak too is behind us, in 2008. Combined oil and gas is expected to have peaked globally simultaneously in 2008.
James Howard Kunstler, in his carefully referenced book The Long Emergency, provides some clues regarding sustainability of our largest cities that goes beyond day-to-day energy consumption. Kunstler predicts that cities like New York will not survive in the post-oil world that is rapidly approaching because of its extreme reliance on food, fuels, goods, and people that are transported from long distances by ship, rail, car, truck, and airplane. Kunstler also points out that skyscrapers and other tall buildings are extremely vulnerable to even fairly short power outages that interrupt heating, air conditioning, water pumps, and elevators. He predicts that such buildings will quickly become uninhabitable as pipes freeze and break and flood units on lower floors. On the one hand dense housing is touted as the vision of the future, and on the other hand there are dire predictions for communities that are not self-sufficient at least in terms of energy, water, and food supplies.
Summary
On average nationwide, dense, multi-family development uses 20-40% less energy for heating and cooling. Engineering analysis indicates that for relatively small multi-family units (1,040 sf), designed to typical energy standards for Boulder County, GHG emission reductions of about 60% are possible compared to heating and cooling of equivalent, single family, detached one-story houses. Dense development also offers potential savings of 10% or more due to reduced vehicle miles traveled because of increased use of alternate transportation modes.
These studies and analyses provide a compelling argument for building with the greatest possible energy efficiency and for powering buildings with renewable energy supplies. Building efficiency considerations also indicate that modest-sized 3 and 4 story units, which the City of Boulder has supported for many years through its Height Ordinance (limiting buildings to 55 ft), are the best choice in terms of GHG emissions compared to taller buildings.
But densification also produces other effects that must be considered, including:
- Embodied energy cannot be ignored. The carbon footprint of embodied energy in materials used for construction is very significant, approaching or even exceeding the energy used in the lifetime operation of the building. Buildings should therefore be built to last using durable, locally-produced materials.
- The external costs of supplying food, fuels, and goods, and traveling to and from communities must be considered in GHG inventories, and they typically aren’t. For Denver, these externalities add an additional 25% to the carbon footprint.
- High-rise buildings have their own set of characteristics, including higher GHG production, very high embodied energy costs, and vulnerability in a post Peak-Oil world.
Whereas it is clear that dense infill development, properly done, can offer real but limited advantages in lower GHG production from heating and cooling compared to detached housing, it is also clear that using a model of population growth to achieve densification will result in a significant increase of our total carbon footprint. Most importantly, buildings should be built to progressively decreasing energy consumption levels, including embodied energy, and should be powered entirely by renewable energy sources. Smaller house size and the external or indirect costs of provisioning dense areas must be part of the solution.
Stay tuned for Part 4: Adequate Public Services
Notes
1. While these authors consider this an advantage, it is generally not considered such. It alters the local climate, sometimes as much as 10 degrees. It creates air conditioning demands that offset heating demand reductions
2. Using the ASHRAE Manual J method for the heat-loss and heat-gain calculations.